Students from Kirschgarten Gymnasium explore AFM technology at Nanosurf, gaining practical insights into nanotechnology ...
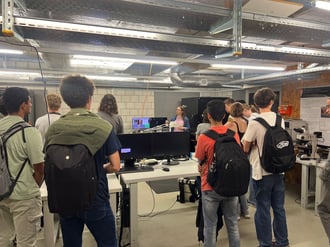
12.06.2024
The ultimate tool for nanoscale research from biological molecules to advanced new materials.
The versatile mid-range research AFM that grows with your demands in modes and accessories.
A compact affordable research AFM that is astoundingly easy to use, with more than 30 modes and options.
Measure roughness and other material properties of heavy and large samples up to 300 mm and 45 kg.
Bringing the power of DriveAFM to a wafer metrology system purpose-built for the requirements of the semiconductor industry.
For unique requirements, we will design a bespoke AFM solution, leveraging our decades of engineering expertise.
Slide an AFM onto your upright optical microscope turret for a leap in resolution.
One of the smallest ever AFMs, created for integration into custom stages or existing setups.
A flexibly mountable research-grade scan head for integration into custom stages or existing set ups.
What is atomic force microscopy (AFM)? How does AFM work? What AFM modes do I really need? How do I get started with AFM?
Learn how AFM works with cantilever/tip assembly interacting with the sample. Explore CleanDrive technology, calibration methods, and feedback principles for precise nanoscale imaging.
An overview of common AFM modes. To learn about each mode in more detail and see application, view the full article.
We regularly publish detailed reviews providing practical guidance and theoretical background on various AFM applications.
Read detailed technical descriptions about selected AFM techniques and learn how to perform specific measurements on Nanosurf instruments.
A library of links to research papers in which Nanosurf instruments were used.
Learn AFM from our library of recorded webinars, covering different measurement techniques, modes, and areas of application.
Short video clips explaining how to perform different operations on Nanosurf instruments.
Watch a product demonstration to learn about the capabilities of our AFMs.
Short videos of our AFMs.
Browse news articles, press releases and a variety of other articles all around Nanosurf.
Browse Héctor Corte-Léon's weekly experiments, for inspiration, entertainment, and to discover everyday applications of AFM.
Héctor here, your AFM expert at Nanosurf calling out for people to share their Friday afternoon experiments. Today I return to one of my favourite topics, memory.
Flash memory chips are ubiquitous since the 80s. Not only on home computers such as the Commodore 64, but also in USB drives, memory cards, hard disk drives (yes, to store the code that makes them tick), toys that play tunes, cameras (I'm talking here about 90s cameras), televisions, and eventually, mobile phones.
The chip I brought here today I found inside of a Kalok hard disk (which I was opening for another fridayAFM). Not sure what it does, it could act as a buffer to read/write onto the hard drive (which makes sense since it has two data banks), or it could act as a storage for the code that controls the hard disk.
Opening the encapsulation is quite easy on these UV-erasable chips, they tend to split in two without too much hassle.
Once opened, a quick inspection with the AFM optical microscope leads to a couple of surprises. First one, despite the Kalok being from 1989... the EPROM design seems to be from 1984 (this reinforces my suspicion that on this hard drive, the bottleneck to higher bit density is due to the way the read/write head is actuated, i.e. with a stepper motor rather than with permanent magnets and coils).
1 Stitched optical images of the IC.
By the way, with the IC designation (HN27C256G according to the encapsulation, HN27C256 according to the die) it is easy to obtain the spreadsheet and details about the chip. It is indeed an EPROM, UV erasable, and once multiplied, the 32768 times 8 bit give us 262144 bits, or 256K.
With the chip open and mounted onto the AFM, and with enough space for the AFM probe to reach almost every corner, I proceeded to take some topography images. The next two images, which are several AFM scans stitched together, show how the memory elements interface with the word and bit decoding structures respectively.
I have absolutely no idea what we see in the decoding area, and couldn't find references for it (other than that the memory is arranged in an array in rows and columns), but the memory element is likely to have the elements highlighted in the diagram. However, the structure I show in the next image is just a guess, based on the visible elements and the requirements of a floating-gate transistor (the "empty" space between elements is supposed to be filled with an insulating barrier).
My guess regarding the transistor shape is based on the fact that the memory elements have a bump in the middle (maybe indicating the pilar going up from the source), and the requirement for the transistor to have three terminals (drain source and gate). In this case, when a voltage is applied to the word line, depending on the charge on the floating gate, it will create an electric field between the bit line and the source pilar that enables (or restricts, depending on the floating gate) the flow of electricity.
So... If the guess with regards to the transistor structure is correct, it means that by measuring surface potential (i.e. doing KPFM), we might be able to see the floating gates, if they still hold any charge.
Ignore the yellow line-shaped signal at the edge of each memory element. It is likely to be either part of the insulation at a much different surface potential, or an artefact due to tip-sample interaction.
From the values of the surface potential (uncalibrated), there is a spread in the potential seen at the centre of the memory elements, which spans from 1.8 to 4V. From a quick glance it is not possible to see a clear division, but if we plot a histogram of the values...
It is clear that there is a gap around 3.3 V, this allow us to categorize the memory elements into two values (i.e. "0" and "1").
So... yes we can retrieve data from this 30+ year old memory device. This also reinforces the argument that this is a pseudo-static random memory, more precisely, a NOR flash memory (the bit line interfaces directly with the memory elements). This is important, because if it were a static memory, it would take four elements to store one bit, while a pseudo-random uses only one. It is critical to establish this point, because of how it compares to our previous fridayAFM. It is curious that as of bit density, this EPROM has smaller bits than a chip from 1985... but they are from different companies... so it is possible that they were using different fabrication processes.
By the way, I hope to fill this plot with more data points in the future, so... stay tuned.
Here it is the previous fridayAFM on another EPROM (also from another hard disk drive).
I hope you enjoyed what I showed you. Stay in touch, and if you have more info, please share (especially if you have information related with the transistor internal geometry).
... and if you made it this far, here are some discarded images.
12.06.2024
Students from Kirschgarten Gymnasium explore AFM technology at Nanosurf, gaining practical insights into nanotechnology ...
29.11.2023
Discover how Nanosurf's collaboration with a team of scientists has revolutionized spring constant calibration for ...
16.10.2023
Discover how Dr. Nitya Nand Gosvami and his team at IIT Delhi harnessed the power of Nanosurf's FlexAFM and DriveAFM ...
08.12.2024
Learn how to make a Python code to interface your AFM with a gamepad.
01.10.2024
FridayAFM: learn how the extreme sensitivity of AFM can reveal the glass ageing process.
11.07.2024
FridayAFM: learn how to perform datamining on large sets of AFM data.
Interested in learning more? If you have any questions, please reach out to us, and speak to an AFM expert.