Discover how Atomic Force Microscopy (AFM) is revolutionizing industries by providing unparalleled insights into ...
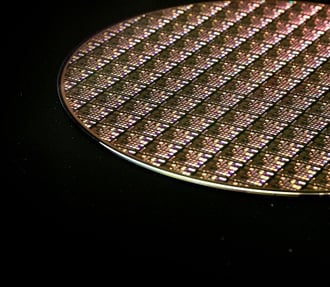
22.10.2024
The ultimate tool for nanoscale research from biological molecules to advanced new materials.
The versatile mid-range research AFM that grows with your demands in modes and accessories.
A compact affordable research AFM that is astoundingly easy to use, with more than 30 modes and options.
Measure roughness and other material properties of heavy and large samples up to 300 mm and 45 kg.
Bringing the power of DriveAFM to a wafer metrology system purpose-built for the requirements of the semiconductor industry.
For unique requirements, we will design a bespoke AFM solution, leveraging our decades of engineering expertise.
Slide an AFM onto your upright optical microscope turret for a leap in resolution.
One of the smallest ever AFMs, created for integration into custom stages or existing setups.
A flexibly mountable research-grade scan head for integration into custom stages or existing set ups.
What is atomic force microscopy (AFM)? How does AFM work? What AFM modes do I really need? How do I get started with AFM?
Learn how AFM works with cantilever/tip assembly interacting with the sample. Explore CleanDrive technology, calibration methods, and feedback principles for precise nanoscale imaging.
An overview of common AFM modes. To learn about each mode in more detail and see application, view the full article.
We regularly publish detailed reviews providing practical guidance and theoretical background on various AFM applications.
Read detailed technical descriptions about selected AFM techniques and learn how to perform specific measurements on Nanosurf instruments.
A library of links to research papers in which Nanosurf instruments were used.
Learn AFM from our library of recorded webinars, covering different measurement techniques, modes, and areas of application.
Short video clips explaining how to perform different operations on Nanosurf instruments.
Watch a product demonstration to learn about the capabilities of our AFMs.
Watch the 8-session short course to get a strong fundamental understanding of AFM.
Browse news articles, press releases and a variety of other articles all around Nanosurf.
Browse Héctor Corte-Léon's weekly experiments, for inspiration, entertainment, and to discover everyday applications of AFM.
Nanoscale imaging is essential in modern research and industry, allowing detailed analysis of materials at atomic and molecular levels. The main techniques are Atomic Force Microscopy (AFM) and Electron Microscopy (EM), which includes Scanning Electron Microscopy (SEM) and Transmission Electron Microscopy (TEM). This article highlights the strengths and limitations of each technique.
AFM uses a sharp probe to scan a sample’s surface, measuring short-ranged interfacial forces to create a quantitative topographic map with high resolution. The probe, typically made of silicon or silicon nitride, interacts with the sample surface through various forces (electrostatic, van der Waals, etc.), causing – in the simplest case – deflection of the cantilever, which is measured by a laser beam. This deflection is used to generate a topographic map with high lateral and vertical resolution, making AFM useful for measuring surface roughness, detecting defects, and characterizing step heights in thin films and nanostructures.
AFM can operate in various environments, including air, vacuum, liquids, and controlled atmospheres, allowing the study of samples in their natural or near-natural states. This versatility is particularly valuable for studying samples that require hydration such as biological materials or that are sensitive to oxygen or humidity. AFM typically requires minimal sample preparation and preserves the sample’s native state. AFM also offers multiple operation modes to characterize local sample properties such as conductivity, surface potential, and stiffness.
SEM uses focused electron beams to scan the sample surface, producing detailed images of surface morphology. When the electron beam interacts with the sample, it generates secondary electrons, backscattered electrons, and characteristic X-rays, which are detected to form an image or provide compositional information. SEM excels in providing detailed images of surface structures with high lateral resolution (1-10 nanometers) and can be combined with techniques like Energy Dispersive X-ray Spectroscopy (EDS) for elemental analysis.
SEM requires samples to be conductive or coated with a thin layer of conductive material to prevent charging under the electron beam. Biological samples may need dehydration and fixation to maintain their structure under vacuum conditions. SEM offers high throughput, making it suitable for rapid imaging over large areas, which is advantageous for quality control in manufacturing processes and screening large numbers of samples in research settings.
TEM transmits electrons through an ultra-thin sample to capture detailed images of internal structures. The electron beam passes through the sample, and interactions within the sample affect the transmitted electrons, forming a 2D projection image or diffraction pattern on a detector. TEM provides atomic-scale resolution (0.1-0.2 nanometers), revealing the arrangement of atoms within the sample, making it ideal for studying crystallography, defects, and the internal structure of materials.
TEM requires extensive sample preparation, including thinning samples to electron transparency (less than 100 nanometers) through techniques like ultramicrotomy or focused ion beam (FIB) milling. This preparation can be time-consuming and may alter the sample’s properties. Despite these challenges, TEM’s unparalleled resolution and detailed imaging capabilities make it a powerful tool for advanced materials research.
Choosing the right nanoscale imaging technique involves considering several key factors, including your research objectives, the nature of your sample, resolution needs, sample preparation tolerance, environmental conditions, and data acquisition requirements.
Criterion | AFM | SEM | TEM |
Resolution |
Vertical Subnanometer Lateral: <1 - 10 nm |
Vertical: no quantitative contrast Lateral: 1-10 nm |
Vertical: No vertical contrast, data analysis may provide 3D structural information Lateral: Sub-nanometer (2D projection of sample) |
Sample Preparation | Minimal | Moderate | Extensive |
Environmental Flexibility | High | Moderate (ESEM for some) | Low (cryo-TEM for some) |
Data Types | Topography, mechanical and electrical properties | Morphology, elemental composition | Internal structure, crystallography |
Acquisition Throughput | Low | High | Low |
Atomic Force Microscopy (AFM) and Electron Microscopy (SEM and TEM) each have distinct advantages and limitations. AFM is known for its high vertical resolution and ability to operate in various environments, making it suitable for topographical and multifunctional measurements, including electrical and nanomechanical properties. In contrast, SEM and TEM offer high lateral resolution and detailed internal structure imaging, respectively. However, they require more extensive sample preparation and operate under vacuum conditions. Researchers should carefully evaluate their specific needs, sample characteristics, and research goals to select the most appropriate nanoscale imaging technique.
22.10.2024
Discover how Atomic Force Microscopy (AFM) is revolutionizing industries by providing unparalleled insights into ...
09.09.2024
Explore the strengths and limitations of Atomic Force Microscopy (AFM), Scanning Electron Microscopy (SEM), and ...
29.11.2023
Discover how Nanosurf's collaboration with a team of scientists has revolutionized spring constant calibration for ...
11.07.2024
FridayAFM: learn how to perform datamining on large sets of AFM data.
02.07.2024
FridayAFM: learn how to automatize data analysis in MountainsSPIP
26.06.2024
FridayAFM: learn how AFM makes possible modern ball point pens.
Interested in learning more? If you have any questions, please reach out to us, and speak to an AFM expert.